Chain of Translatability
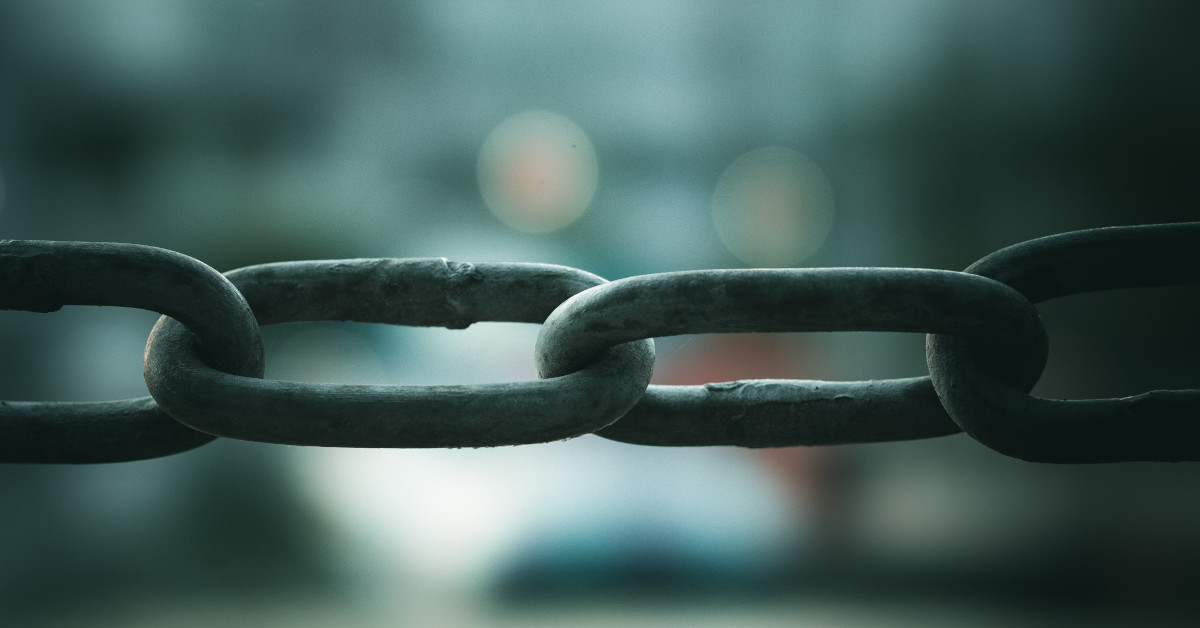
A strong chain of translatability is crucial for successful drug discovery programs. The chain of translatability is a molecular-level association between the mechanisms that drive the assay phenotype, the preclinical disease model and the human disease. Can we build stronger chains with better preclinical disease models?
Don Ingber raises this point in his recent article, “Is it Time for Reviewer 3 to Request Human Organ Chip Experiments Instead of Animal Validation Studies?”
Reviewers should indeed resist the reflexive response of asking for animal studies to support clinical relevance of in vitrofindings. Animals are not mini people.
Most biomedical researchers simply do not appreciate just how misaligned animal models are with human diseases. Ingber points out a few examples in his article, mentioning clinical failures in ARDS (acute respiratory distress syndrome) and Alzheimer’s disease. We have previously discussed the impact of differences in lifespan, environment and physical architecture on mechanisms of toxicity and drug discovery in oncology, inflammatory and cardiovascular disease (and see also this post).
So what to do?
Are organ chips the answer? Can they replace animal studies? Perhaps in part, but not entirely.
Ingber describes a variety of in vitro alternatives to animals: organoids, microphysiological systems, organ chips and multi-organ chips. He presents a review of valuable discoveries and applications of these technologies. These include uncovering mechanisms underlying drug pharmacodynamic behaviors, distinguishing species specific toxicities, finding new insights into rare genetic disorders, supporting target validation and discovering the role of fluid flow and mechanical forces on organ function.
For drug discovery applications, the success stories using organ chips, as wonderful as they are, remain anecdotal. Few studies evaluate more than one or two drugs. Most are individual case studies, illustrating the successful replication of some clinical observation. They do not constitute a systematic assessment of predictivity nor do they establish generalizability. The volume of data needed for this is unlikely to be generated any time soon. Organ chips have limited throughput and can be variable (requiring high numbers of replicates). In addition, research efforts continue to be directed towards developing new models or improving current models. Ingber himself articulates a desire to include even more features of human physiology into existing models.
So in the absence of large-scale validation testing, how can we gain confidence in the use of these technologies?
One approach that may be helpful is to integrate organ chip experiments more tightly with supportive mechanism-based assays. The use of knowledge-based decision support frameworks can facilitate this. These frameworks include adverse outcome pathways (AOPs) and discovery program outcome pathways (DPOPs) used in chemical risk assessment and drug discovery program management. They are used to connect experimental results from assays at different levels of biological complexity through mechanistic connections. Assays can include high throughput assays, target screens, pathway mechanistic-based screens, cell-based phenotypic assays, organoids, tissue chips, and clinical outcome studies. Logical connections between knowledge at the molecular level through the cell and tissue levels and to patient outcomes are used to support the “chain of translatability”.
Organ chips are ideally suited for bridging the gap between research and development. Being human they are more easily connected to molecular and pathway assays as well as to clinical measures.
Now how we make this happen is the next challenge.
Photo credit: Daniel von Appen on Unsplash